The Webster dictionary defines a tide as “the alternate rising and falling of the surface of the ocean that occurs twice a day and is caused by the gravitational attraction of the sun and moon occurring unequally on different parts of the earth”.
Tide changes proceed via the following stages
- Sea level rises over several hours, covering the intertidal zone; flood tide.
- The water rises to its highest level, reaching high tide.
- Sea level falls over several hours, revealing the intertidal zone; ebb tide.
- The water stops falling, reaching low tide.
- Tides produce oscillating currents known as tidal streams. The moment that the tidal current ceases is called slack water or slack tide. The tide then reverses direction and is said to be turning. Slack water usually occurs near high water and low water. But there are locations where the moments of slack tide differ significantly from those of high and low water.
Tides are commonly semi-diurnal (two high waters and two low waters each day), or diurnal (one tidal cycle per day). The two high waters on a given day are typically not the same height (the daily inequality); these are the higher high water and the lower high water in tide tables. Similarly, the two low waters each day are the higher low water and the lower low water. The daily inequality is not consistent and is generally small when the Moon is over the equator.
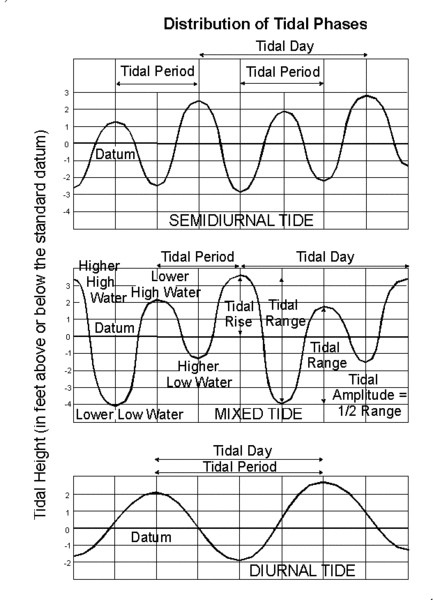
Tidal Range
Tidal range is the vertical difference between high and low tide.
The power that can be captured from water flowing from high to low is proportional to the squared height (or head h):
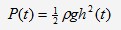
with ρ = 1,023kg/m3 and acceleration g=9.81m/s2.
Only sites with sufficient head can be used for commercial purposes.
The 50 sites with the highest tidal ranges in the world are in just 6 regions
- Bay of Fundy, Canada with up to 12m mean tide
- Bristol Channel and Cardiff Bay, UK
- Normandy, France
- Magellan Strait, Argentina & Chile
- Cook Inlet, Alaska, USA
- Penzhinskaya Bay, Kamtchatka, Russia
Challenges
- Most sites are in remote areas that cannot consume all the energy. Strengthening of the grid is required.
- Environmental aspect: How much energy is acceptable to be taken out of a particular site? Unlike in the wind energy industry, there is no established process for environmental impact analysis.
- New technology developers will need to proof that their devices are significantly more efficient, robust or cheaper to operate.
- The market may be limited to a small number of countries.
- Requirements for navigational clearance may hinder installations.
Opportunities
- Largely untapped source with fairly low technology risk and synergies with off-shore wind industry.
- Unlike solar, tidal stream energy lends itself to clusters of large-scale projects, a business model favoured by utility companies.
- As tidal energy is uncorrelated to wind energy, tidal capacity leads to an increase in the total capacity credit of a renewable energy portfolio, and therefore a reduction in required fossil back-up capacity. This could be as much as 3.3% cost saving per kWh (according to Carbon Trust).
- High capacity factors of > 40% help make the technology economically competitive.
Tidal Energy Estimation
The energy available from barrage is dependent on the volume of water. The potential energy contained in a volume of water is:
E=1/2 Apgh2
where:
h is the vertical tidal range,
A is the horizontal area of the barrage basin,
ρ is the density of water = 1025 kg per cubic meter (seawater varies between 1021 and 1030 kg per cubic meter) and
g is the acceleration due to the Earth’s gravity = 9.81 meters per second squared.
The factor half is due to the fact, that as the basin flows empty through the turbines, the hydraulic head over the dam reduces. The maximum head is only available at the moment of low water, assuming the high water level is still present in the basin.
Energy Potential Estimation For A Tidal Power Plant
Simulation Model
The model determines tide levels at 6-minute intervals for any day, month, or year for the period of years between 1949 and 2025. The tide levels are predicted from a series of harmonic equations as provided in a FORTRAN program developed by NOAA, in the case of US sites or by the British Admiralty, in the case of British sites. Predicted tide levels may vary from actual tide levels due to weather conditions such as wind and atmospheric pressure. A comparison of tide levels predicted by the model and actual recorded tide levels showed agreement well within acceptable limits for a feasibility study. The constantly changing tidal regime would cause generation to vary by several percent from year to year. Other things being equal, it was found that during a high generation year (1998) project output would be about 8% greater than in a low generation year (2005). This cycle is known as the nodal cycle.
A hypothetical equipment package is selected and its operating parameters are input and an impoundment size is selected, which defines the amount and timing of water available and the sequence of heads available. Together, these parameters determine the turbine flow and efficiency as well as the generator efficiency. Because the turbines have minimal heads, there are periods of sluicing that are essential to the operation in order to maximize or minimize the water levels in the pool in preparation for the next generation cycle. Some of the sluicing flows through the turbines and sluice gates provide addition sluicing capacity.
Sluicing is determined from the following equation:
Q = C*A*(2g*H) 1/2
Where:
Q is the sluice flow in cfs
C is the sluice gate coefficient or turbine sluicing
coefficient A is the sluice gate area (ft2) or turbine area for sluicing (ft2)
H is the head on the sluice (feet) g is 32.2 ft/sec2
The net energy is determined by using the following formula
E = 0.08464*Q*H*Et*Eg*Etr*Lt*Lsu*Lo*T
Where:
E is the energy generation in kilowatt-hours
Q is the turbine flow in cfs
H is the net head on the turbine in feet
Et is the turbine efficiency
Eg is the generator efficiency
Etr is the transformer efficiency
Lt is the transmission line loss factor
Lsu is the station use loss factor
Lo is the forced and unforced outage loss factor
T is the time increment (0.1 hour in the model)
Hydraulic losses unavoidably occur near the intake and outlet of a turbine. These head losses are included based on the following equation
HL = k*Q2
Where:
HL is the head loss in feet
k is the head loss coefficient
Q is the turbine flow in cfs
Transmission line losses are included to the point of interconnection where the sale of electricity is metered. Some electricity use normally occurs at the project site, which is accounted for in the station use factor. The forced outage loss factor should account for average outages of all types over the economic life of the project. Outage losses would include factors such as transmission line forced outages, and down time for equipment repair, both scheduled and unscheduled.
Turbine Power
Tidal energy converters can have varying modes of operating and therefore varying power output. If the power coefficient of the device “” is known, the equation below can be used to determine the power output of the hydrodynamic subsystem of the machine. This available power cannot exceed that imposed by the Betz limit on the power coefficient, although this can be circumvented to some degree by placing a turbine in a shroud or duct. This works, in essence, by forcing water which would not have flowed through the turbine through the rotor disk. In these situations it is the frontal area of the duct, rather than the turbine, which is used in calculating the power coefficient and therefore the Betz limit still applies to the device as a whole. The energy available from these kinetic systems can be expressed as
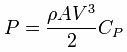
where:
= the turbine power coefficient
P = the power generated (in watts)
= the density of the water (seawater is 1027 kg/m³)
A = the sweep area of the turbine (in m²)
V = the velocity of the flow
Relative to an open turbine in free stream, ducted turbines are capable of as much as 3 to 4 times the power of the same turbine rotor in open flow.
Resource assessment
While initial assessments of the available energy in a channel have focus on calculations using the kinetic energy flux model, the limitations of tidal power generation are significantly more complicated. For example, the maximum physical possible energy extraction from a strait connecting two large basins is given to within 10% by

where
= the density of the water (seawater is 1027 kg/m³)
g = gravitational acceleration (9.80665 m/s2)
= maximum differential water surface elevation across the channel
= maximum volumetric flow rate though the channel.
Energy and Power In A Double Cycle System
Power is generated while filling the basin and also while emptying the basin. Hence double energy scheme gives double energy per tide cycle of 1 crest and 1 ebb.
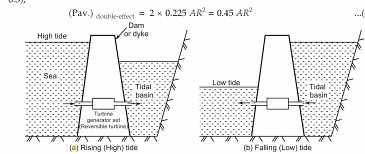
Yearly Power Generation From Tidal Plants
The tidal energy derived from a tidal plant depends on the following two factors
- Tidal range
- Amount of water accumulated in the basin
Tidal energy is slowly filling hydro energy during the filling of the basin and after a period of 3 hours, it reaches it’s peak value. When the tide recedes, water is allowed to flow from the basin to the sea: it is then slowly decreasing hydro energy and it attains it’s lowest value after the turbine stops after a period of 3 hours. The energy available at a tidal point at any instant can be calculated by considering the average discharge and the available head at any instant.
Tidal Energy Generation
Tidal power is taken from the Earth’s oceanic tides; tidal forces are periodic variations in gravitational attraction exerted by celestial bodies. These forces create corresponding motions or currents in the world’s oceans. Due to the strong attraction to the oceans, a bulge in the water level is created, causing a temporary increase in sea level. When the sea level is raised, water from the middle of the ocean is forced to move toward the shorelines, creating a tide. This occurrence takes place in an unfailing manner, due to the consistent pattern of the moon’s orbit around the earth. The magnitude and character of this motion reflects the changing positions of the Moon and Sun relative to the Earth, the effects of Earth’s rotation, and local geography of the sea floor and coastlines.
Tidal power is the only technology that draws on energy inherent in the orbital characteristics of the Earth–Moon system, and to a lesser extent in the Earth–Sun system. Other natural energies exploited by human technology originate directly or indirectly with the Sun, including fossil fuel, conventional hydroelectric, wind, biofuel, wave and solar energy. Nuclear energy makes use of Earth’s mineral deposits of fissionable elements, while geothermal power taps the Earth’s internal heat, which comes from a combination of residual heat from planetary accretion (about 20%) and heat produced through radioactive decay (80%).
A tidal generator converts the energy of tidal flows into electricity. Greater tidal variation and higher tidal current velocities can dramatically increase the potential of a site for tidal electricity generation.
Because the Earth’s tides are ultimately due to gravitational interaction with the Moon and Sun and the Earth’s rotation, tidal power is practically inexhaustible and classified as a renewable energy resource. Movement of tides causes a loss of mechanical energy in the Earth–Moon system: this is a result of pumping of water through natural restrictions around coastlines and consequent viscous dissipation at the seabed and in turbulence. This loss of energy has caused the rotation of the Earth to slow in the 4.5 billion years since its formation. During the last 620 million years the period of rotation of the earth (length of a day) has increased from 21.9 hours to 24 hours; in this period the Earth has lost 17% of its rotational energy. While tidal power will take additional energy from the system, the effect is negligible and would only be noticed over millions of years.
Generating Methods
Tidal power can be classified into four generating methods:
Tidal stream generator – Tidal stream generators (or TSGs) make use of the kinetic energy of moving water to power turbines, in a similar way to wind turbines that use wind to power turbines. Some tidal generators can be built into the structures of existing bridges, involving virtually no aesthetic problems. Land constrictions such as straits or inlets can create high velocities at specific sites, which can be captured with the use of turbines. These turbines can be horizontal, vertical, open, or ducted and are typically placed near the bottom of the water column.
Tidal barrage – Tidal barrages make use of the potential energy in the difference in height (or hydraulic head) between high and low tides. When using tidal barrages to generate power, the potential energy from a tide is seized through strategic placement of specialized dams. When the sea level rises and the tide begins to come in, the temporary increase in tidal power is channeled into a large basin behind the dam, holding a large amount of potential energy. With the receding tide, this energy is then converted into mechanical energy as the water is released through large turbines that create electrical power through the use of generators. Barrages are essentially dams across the full width of a tidal estuary.
Dynamic tidal power – Dynamic tidal power (or DTP) is an untried but promising technology that would exploit an interaction between potential and kinetic energies in tidal flows. It proposes that very long dams (for example: 30–50 km length) be built from coasts straight out into the sea or ocean, without enclosing an area. Tidal phase differences are introduced across the dam, leading to a significant water-level differential in shallow coastal seas – featuring strong coast-parallel oscillating tidal currents such as found in the UK, China, and Korea.
Tidal lagoon – A newer tidal energy design option is to construct circular retaining walls embedded with turbines that can capture the potential energy of tides. The created reservoirs are similar to those of tidal barrages, except that the location is artificial and does not contain a preexisting ecosystem. The lagoons can also be in double (or triple) format without pumping or with pumping that will flatten out the power output. The pumping power could be provided by excess to grid demand renewable energy from for example wind turbines or solar photovoltaic arrays. Excess renewable energy rather than being curtailed could be used and stored for a later period of time. Geographically dispersed tidal lagoons with a time delay between peak production would also flatten out peak production providing near base load production though at a higher cost than some other alternatives such as district heating renewable energy storage.
Components of A Tidal Power Plant
There are many components of a tidal power plant, which are
Dam: a barrier constructed to hold back water and raise its level, forming a reservoir used to generate electricity or as a water supply.
A barrage: It is a small wall built at the entrance of a gulf in order to trap water behind it. It will either trap it by keeping it from going into the gulf when water levels at the sea are high or it will keep water from going into the sea when water level at the sea is low.
Sluice ways: an artificial water channel for carrying off overflow or surplus water. Sluice gates are the ones responsible for the flow of water through the barrage.
Power house: A powerhouse has turbines, electric generators and other auxiliary equipment. As far as possible, the powerhouse should be in alignment with the dam or dyke.
Embankments: They are caissons made out of concrete to prevent water from flowing at certain parts of the dam and to help maintenance work and electrical wiring to be connected or used to move equipment or cars over it.
Turbines: They are the components responsible for converting potential energy into kinetic energy. They are located in the passageways that the water flows through when gates of barrage are opened. There are many types of turbines used in tidal power stations, which are
- Bulb turbines: These are difficult to maintain as water flows around them and the generator is in water.
- Rim turbines: These are better maintained than the bulb turbines but are hard to regulate as generator is fixed in barrage.
- Tabular turbines: These turbines are fixed to long shafts and thus solve both problems that bulb and rim turbines have as they are easier to maintain and control.
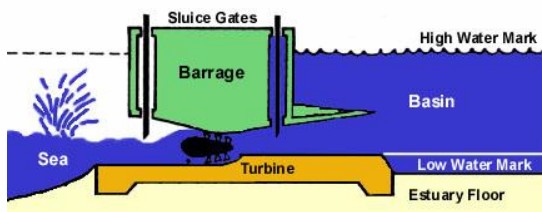
Grid Interfacing of Tidal Power
The energy harnessed in the form of mechanical energy, is converted to electrical energy with the help of auxiliary equipment such as submarine cables, a protection system designed accordingly and the electrical networks including various connections and earthing.
Advantages And Disadvantages of Tidal Power
Advantage of tidal power energy
- Renewable resource, it needs no fuel to maintain, and free of charge
- Totally no pollution, unlike fossil fuels, it produces no greenhouse gases or other waste.
- Predictable source of energy (compared with wind and solar), it is independent of weather and climate change and follows the predictable relationship of the lunar orbit
- More efficient than wind because of the density of water
- It will protect a large stretch of coastline against damage from high storm tides
Disadvantage of tidal power energy
- Presently costly, very expensive to build and maintain (A 1085MW facility could cost about 1.2 billion dollars to construct and run)
- Barrage has environmental affects a) fish and plant migration b) Silt and mud deposits c) Waste and sewage blocks
- Technology is not fully developed
- Only provides power for around 10 hours each day, when the tide is actually moving in or out
Economics of Tidal Power
Even though Tidal barrage power schemes have a high capital cost they also have a very low running cost. Whilst a tidal power scheme may not generate returns for many years, once it moves into surplus it is likely to go on providing a profit for an indefinite, long period. Because of the long term nature of returns, private investors may be reluctant to participate, and central governments may have to lead the way.
Tidal Power Development In India
India has always played an active role in researching and developing new the technologies. The main objective of the tidal energy programme executed by the Ministry of New and Renewable Energy, Govt. of India is to study, testing and assessment of the potential of tidal energy in the country and to harness it for power generation. Among the various forms of energy contained in the seas and oceans, tidal energy, has been developed on a commercial scale. India has a long coastline with the estuaries and gulfs where tides are strong enough to move turbines for electrical power generation. The Gulf of Cambay and the Gulf of Kutch in Gujarat on the west coast have the maximum tidal range of 11m and 8m with average tidal range of 6.77m and 5.23m respectively.
The Ganges Delta in the Sundarbans is approximately 5m with an average tidal range of 2.97m. The identified economic power potential is of the order of 8000 MW with about 7000 MW in the Gulf of Cambay, about 1200 MW in the Gulf of Kutch in the State of Gujarat and about 100 MW in the Gangetic Delta in the Sunderbans region in the State of West Bengal. The Ministry sanctioned a project for setting up a 3.75 MW demonstration tidal power plant at Durgaduani Creek in Sunderbans, West Bengal to the West Bengal Renewable Energy Development Agency (WBREDA), Kolkata. The National Hydro Power Corporation Ltd. (NHPC) is executing the project on a turnkey basis. The State Government of Gujarat formed a Special Purpose Vehicles (SPVs) with public private partnership and sponsored a study for large scale exploitation of tidal energy across the coastline of Gujarat. This study is based on one of the advanced technologies developed so for. In this technology kinetic energy of tidal currents has been proposed to be harnessed under the water and along the flow of water and without using the conventional methods like water wheel or other types of turbines. Also the state of Gujarat is ready to host Asia’s first commercial based tidal power station. The company Atlantis Resources Corporation is to install a 50MW tidal farm in the Gulf of Kutch on India’s west coast, with the construction starting early this year. The company has signed a MoU with the government of Gujarat to set up the power plant by 2013. The plant is likely to be scaled up to a capacity of 250 MW at a cost of about $165 million. The project will be developed in partnership with the Gujarat Power Corporation Limited