According to Muffler and Cataldi (1978), when we speak generically about geothermal resources, what we are usually referring to is what should more accurately be called the accessible resource base; that is, all of the thermal energy stored between the Earth’s surface and a specified depth in the crust, beneath a specified area and measured from local mean annual temperature. The accessible resource base includes the useful accessible resource base (= Resource ) — that part of the accessible resource base that could be extracted economically and legally at some specified time in the future (less than a hundred years). This category includes the identified economic resource (= Reserve ) — that part of the resources of a given area that can be extracted legally at a cost competitive with other commercial energy sources and that are known and characterised by drilling or by geochemical, geophysical and geological evidence. Figure below illustrates in graphic form these and other terms that may be used by geothermal specialists.
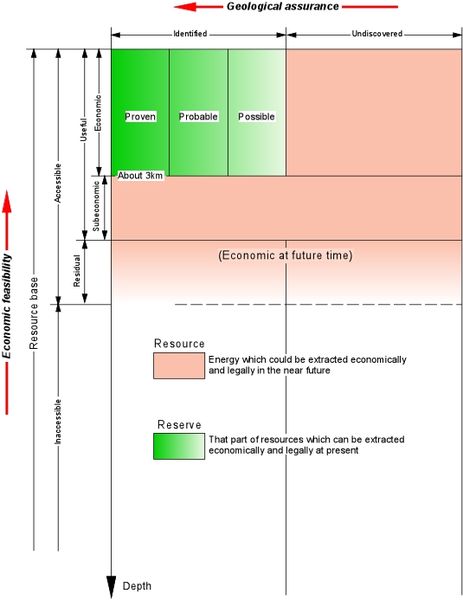
The most common criterion for classifying geothermal resources is, however, that based on the enthalpy of the geothermal fluids that act as the carrier transporting heat from the deep hot rocks to the surface. Enthalpy, which can be considered more or less proportional to temperature, is used to express the heat (thermal energy) content of the fluids, and gives a rough idea of their ‘value’. The resources are divided into low, medium and high enthalpy (or temperature) resources, according to criteria that are generally based on the energy content of the fluids and their potential forms of utilization. Table 3 reports the classifications proposed by a number of authors. A standard method of classification, as with terminology, would avoid confusion and ambiguity but, until such a method exists, we must indicate the temperature values or ranges involved case by case, since terms such as low, intermediate and high are meaningless at best, and frequently misleading.
Frequently a distinction is made between water- or liquid-dominated geothermal systems and vapour-dominated (or dry steam) geothermal systems (White, 1973). In water-dominated systems liquid water is the continuous, pressure-controlling fluid phase. Some vapour may be present, generally as discrete bubbles. These geothermal systems, whose temperatures may range from < 125 to > 225 °C, are the most widely distributed in the world. Depending on temperature and pressure conditions, they can produce hot water, water and steam mixtures, wet steam and, in some cases, dry steam. In vapour-dominated systems liquid water and vapour normally co-exist in the reservoir, with vapour as the continuous, pressure-controlling phase. Geothermal systems of this type, the best-known of which are Larderello in Italy and The Geysers in California, are somewhat rare, and are high-temperature systems. They normally produce dry-to- superheated steam.
The terms wet, dry and superheated steam, which are used frequently by geothermists, need some explanation for readers who are not of an engineering background. To make it as simple as possible, let us take the example of a pot filled with liquid water in which pressure can be kept constant at 1 atm (101.3 kPa). If we then heat the water, it will begin boiling once it reaches a temperature of 100 °C (boiling temperature at a pressure of 1 atm) and will pass from the liquid to the gas (vapour) phase. After a certain time the pot will contain both liquid and vapour. The vapour coexisting with the liquid, and in thermodynamic equilibrium with it, is wet steam. If we continue to heat the pot and maintain the pressure at 1 atm, the liquid will evaporate entirely and the pot will contain steam only. This is what we call dry steam. Both wet and dry steam are called “saturated steam”. Finally, increasing the temperature to, say, 120 °C, and keeping the pressure at 1 atm, we will obtain superheated steam with a superheating of 20 °C, i.e. 20 °C above the vaporisation temperature at that pressure. At other temperatures and pressures, of course, these phenomena also take place in the underground, in what one author many years ago called “nature’s tea-kettle”.
Another division between geothermal systems is that based on the reservoir equilibrium state (Nicholson, 1993), considering the circulation of the reservoir fluid and the mechanism of heat transfer. In the dynamic systems the reservoir is continually recharged by water that is heated and then discharged from the reservoir either to the surface or into underground permeable formations. Heat is transferred through the system by convection and circulation of the fluid. This category includes high-temperature (>150 °C) and low-temperature (<150 °C) systems. In the static systems(also known as stagnant or storage systems) there is only minor or no recharge to the reservoir and heat is transferred only by conduction. This category includes low-temperature and geopressured systems. The geopressured systems are characteristically found in large sedimentary basins (e.g. Gulf of Mexico, USA) at depths of 3 – 7 km. The geopressured reservoirs consist of permeable sedimentary rocks, included within impermeable low-conductivity strata, containing pressurized hot water that remained trapped at the moment of deposition of the sediments. The hot water pressure approaches lithostatic pressure, greatly exceeding the hydrostatic pressure. The geopressured reservoirs can also contain significant amounts of methane. The geopressured systems could produce thermal and hydraulic energy (pressurized hot water) and methane gas. These resources have been investigated extensively, but so far there has been no industrial exploitation.